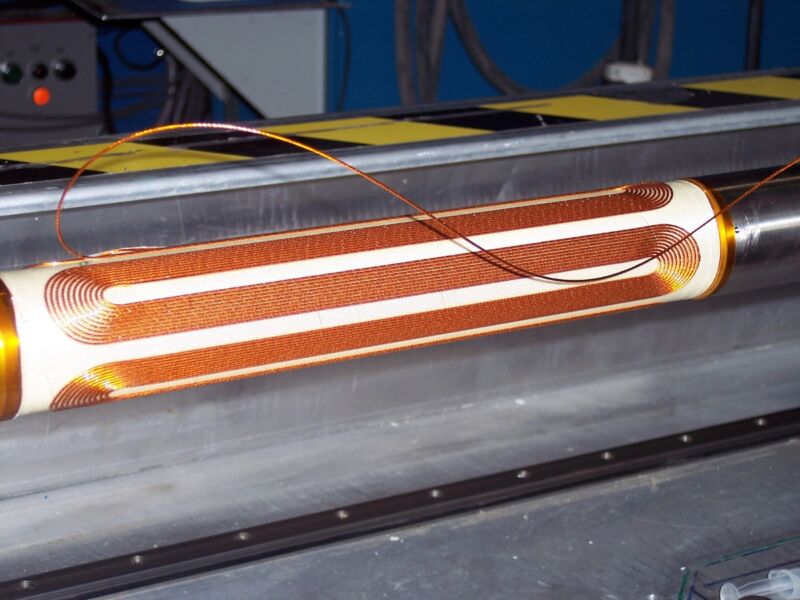
On Wednesday, scientists reported successfully cooling atoms made of antimatter using an ultraviolet laser. The cooling process deployed here works on normal matter, so it was also expected to work just fine with the antihydrogen used for these experiments. Rather than providing yet another confirmation that matter and antimatter appear to behave the same, the experiment is significant because it increases our chances of being able to identify subtle differences between the two types of matter.
Exotic yet apparently normal
Normal matter and antimatter are notable for their ability to annihilate each other in a burst of energy when they come in contact. But otherwise, our understanding of the physics of antiparticles indicates that they should behave identically—an antiproton can pair with an antielectron to form antihydrogen, which would then be subject to forces like gravity and electromagnetism. As far as most of physics is concerned, antimatter is just matter reflected by a mirror: a few things reversed, but otherwise identical.
Testing that, however, is a challenge. All the antimatter produced naturally on Earth comes from energetic processes like radioactive decays and the impact of cosmic rays. As a result, the antimatter itself carries plenty of energy, and it moves very quickly. Similar things apply to antimatter we humans produce. It's generally made by colliding energetic particles with a stationary target, and the antimatter that comes out of these collisions is also very energetic.
Making rough measurements of this antimatter is possible, despite its high energies, and those measurements have confirmed that it behaves the same way that matter would (excepting its energetic end once it interacts with matter). But if there are more subtle differences, we wouldn't see them. Because the antimatter is in motion, the measurements have substantial errors that would obscure any subtleties.
It's only been in the past decade or so that we've built the hardware needed in order to get antimatter to slow down enough to form atoms. There's now dedicated hardware at CERN that slows down antiprotons, combines them with antielectrons, and then stores the resulting antimatter for study.
But the atoms trapped by that system end up having a substantial amount of energy. If you tried to figure out the energy involved in shifting the antielectron between orbitals using photons, the measurement would result in a broad curve rather than a sharp peak. That's because some of the atoms would be moving toward the photon source while others moved away from it, resulting in Doppler shifts that alter the wavelengths that get absorbed.
Shift to chill
Fortunately, we have no shortage of experience at cooling atoms down to where they carry very little energy. One of the most effective methods involves using the Doppler effect as well.
The approach works because photons, while having no mass, carry momentum. So, when they're absorbed by an atom, the atom picks up some of the momentum. However, if you shine a laser at a wavelength that atoms absorb, on average, all the momentum changes wash out. That's because the added momentum will speed up some atoms and slow down others.
The trick to using this method to cool things down involves picking a laser with a wavelength that's slightly redder than the ones the atoms would typically absorb. Most of the photons would simply pass through the atoms, since they're the wrong wavelength. But atoms that are moving toward the photon source will see the wavelength shift slightly toward the blue end of the spectrum. Since the photons are too red, this shifts them into being just the right wavelength.
As a result, only the atoms that are moving toward the photon source get slowed down. And none of them ends up getting sped up. Over time, the net speed of the atoms will drop, cooling the sample down.
The wavelength needed for the antihydrogen (it would also work on hydrogen) turned out to be inconveniently in the UV. To hit the right wavelength, the researchers had to start with a laser with a much longer wavelength and increase the photons' energy through two separate processes. Each of these processes loses energy, so the resulting laser was pretty weak.
That's a problem, because each of the antihydrogen atoms needs to absorb a lot of photons. Each atom starts out with a typical speed of about 90 meters/second, and a photon only knocks 3.3 meters/second off that. In this case, the researchers made up for the relatively low efficiency with time. The CERN setup fills its storage area over the course of hours in short bursts of antiatom deliveries. Between each of these bursts, the laser is able to cool down the atoms already there.
It’s chill
Fortunately, the CERN setup is so good at storing antimatter that the researchers were able to run experiments on it that lasted over 17 hours. And, during that time, the cooling reduced the signal from antimatter annihilations in the setup. This confirmed that the laser cooling was reducing the overall energy and thus reducing the number of antiatoms with enough energy to escape the containment.
And it worked. The researchers used a laser that was too blue as a control, and then it excited the antihydrogen's antielectron into a new orbital. This experiment produced the sort of broad peak you'd expect from a population of atoms in motion. Using a laser at the correct, slightly redder wavelength saw that peak narrow considerably, indicating a population of atoms that was not moving as quickly.
The data included a small surprise. Because of all the magnets and other equipment needed to trap antimatter without it running into actual matter, the cooling laser only entered the trap in one direction. This should lead to slowing the antiatoms down only along that axis. But the atoms seemed to slow down in all directions. The researchers ascribe this to the complicated magnetic fields needed to contain the antimatter, which couples motion in any direction to any other direction.
But this work really isn't about producing surprises. Instead, it's meant to enable the sorts of work where surprises might appear. With the chilled antihydrogen, researchers can now perform much more precise measurements, allowing more subtle differences between matter and antimatter to become apparent. It's possible that we have physics right and those difference won't be there. But it would be really annoying if there was a difference that we didn't check.
Nature, 2021. DOI: 10.1038/s41586-021-03289-6 (About DOIs).
"Give" - Google News
April 01, 2021 at 05:45PM
https://ift.tt/3rESewj
Physicists give antimatter the chills - Ars Technica
"Give" - Google News
https://ift.tt/2YqGX80
https://ift.tt/2YquBwx
Bagikan Berita Ini
0 Response to "Physicists give antimatter the chills - Ars Technica"
Post a Comment